A closer look at dust particles
Young planet-forming disks contain trillions of tiny microscopic dust particles. Even in the tenuous protoplanetary disk, these particles bump into each other every now and then, sticking together and growing larger and larger with every collision, eventually forming the building blocks of planets. Historically, dust grains have been treated as being spherical and compact, but in recent years the evolution of the internal structure (or, porosity) of these particles has received more and more attention – and for good reason.
Hit-and-stick Tetris
For dust particles smaller than ~1 millimeter, typical collision velocities in protoplanetary disks are below 1 cm/s. At these low velocities, collisions are in the so-called “hit-and-stick” regime: particles stick together upon contact without any restructuring taking place (the sticking is made possible by Van der Waals forces, the same forces that allow geckos to climb walls). A good analog for hit-and-stick growth in 2 dimensions is Tetris.
Figure 1 shows three outcomes of Tetris games where I was trying to mimic random impacts by playing the game with my eyes closed. The results show that undirected hit-and-stick growth naturally leads to a porous structure, filled with voids and gaps of various sizes. The average porosity (calculated as the fraction of space not taken up by solids) is very close to 50% for all three panels. This 50% is by no means a universal number and depends for example on the properties of the particles that we are adding from the top. In classic Tetris, all building blocks are composed of 4 squares (hence the name), but if we were to repeat the experiment with single squares raining down, the final porosity would be effectively 0%.
Porosity evolution in protoplanetary disks
Dust aggregates growing in protoplanetary disks follow similar rules: hit-and-stick growth leads to porous (or “fluffy”) aggregates, with the degree of porosity depending on the sizes and shapes of the collision partners. Figure 2 shows two dust particles grown in 3D computer simulations using different assumptions about the distribution of collision partners. Both aggregates have a final mass of ~0.1 microgram, but their sizes, structures, and (average) porosities are very different.
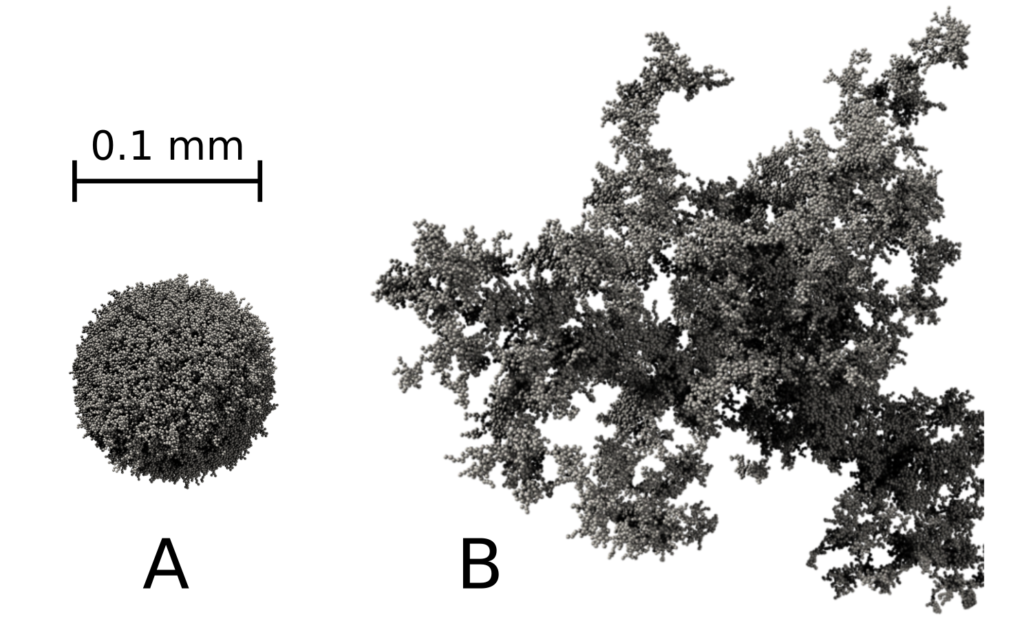
Fig. 2: Two dust aggregates grown in computer simulations. Aggregate A has grown by many hit-and-stick collisions with micrometer-size grains, resulting in an average porosity of 80%. Aggregate B has grown by colliding with particles similar to itself, which is closer to what is expected to occur in protoplanetary disks, and has a porosity >99% (from: Seizinger, Krijt, and Kley – Astronomy & Astrophysics, 2013).
As aggregates grow larger still, collisions become increasingly energetic and instead of hitting-and-sticking they can result in significant compaction (i.e., the crushing of voids, or “de-fluffification”) or even (partial) disruption of the aggregates. The collisional outcomes and porosity evolution in this more energetic regime are very complex and understanding both requires an intimate knowledge of how different aggregates respond to mechanical stresses. Laboratory experiments and numerical simulations agree to some extent (for example, both are finding that aggregates consisting of ice-coated grains are harder to compress and destroy than rocky ones), but large regions of parameter space are still unexplored: simulations are restricted to relatively small particle sizes and experiments have difficulty probing the highly-porous regime.
One thing appears clear though: dust aggregates are unlikely to be spherical, compact, and of a uniform density. With porosity profoundly influencing an individual particle’s optical, mechanical, and aerodynamical properties, developing a more realistic model for the particle’s structure is very important for astronomers studying the appearance and evolution of dust in planet-forming disks, and remains an area of ongoing research.