Module One: The Conditions and Timeline for Planet Formation
Protoplanetary disks are the sites of planet formation and the source of planetary building blocks. Over the past few years, sub-millimeter imaging has revealed that disk structures are common and more complex than anticipated, necessitating new, advanced studies of protoplanetary disks.
Methods
Protoplanetary Disk Observations
Planet Formation and Disk Evolution Models
Cosmochemical Studies of Meteorites and Returned Samples
The Early Evolution of Planet-forming Disks
Project 1.1 | Lead: Josh Eisner
The properties of protoplanetary disks, including their dust and gas masses, compositions, and holes, gaps, or other structures, constrain the mechanisms by which planets can form. Most studies of these disks have targeted evolved “Class II” disks. Many of these systems, which are aged 1–10 Myr, show evidence of dust sub-structures, suggesting ongoing planet formation. However, planet formation may be underway even before the Class II stage, as evinced by (potentially) planet-induced structure observed in younger “Class I” protostars. Targeting these Class I systems for detailed study will provide a clearer picture of the pristine planet-forming environment. Indeed, Class I systems show higher dust masses, indicating less processing into large bodies at that stage, as well as smaller disk sizes associated with less viscous spreading. As disk evolution also depends on stellar mass, we must seek a comprehensive understanding of the target samples compared.
In Project 1.1 we will extend our ongoing ALMA sub-mm surveys to quantify the distribution and evolution of disk masses (both the dust and the gas components) from Class I (embedded) disks to Class II disks, using data available from our EOS project and from the literature.
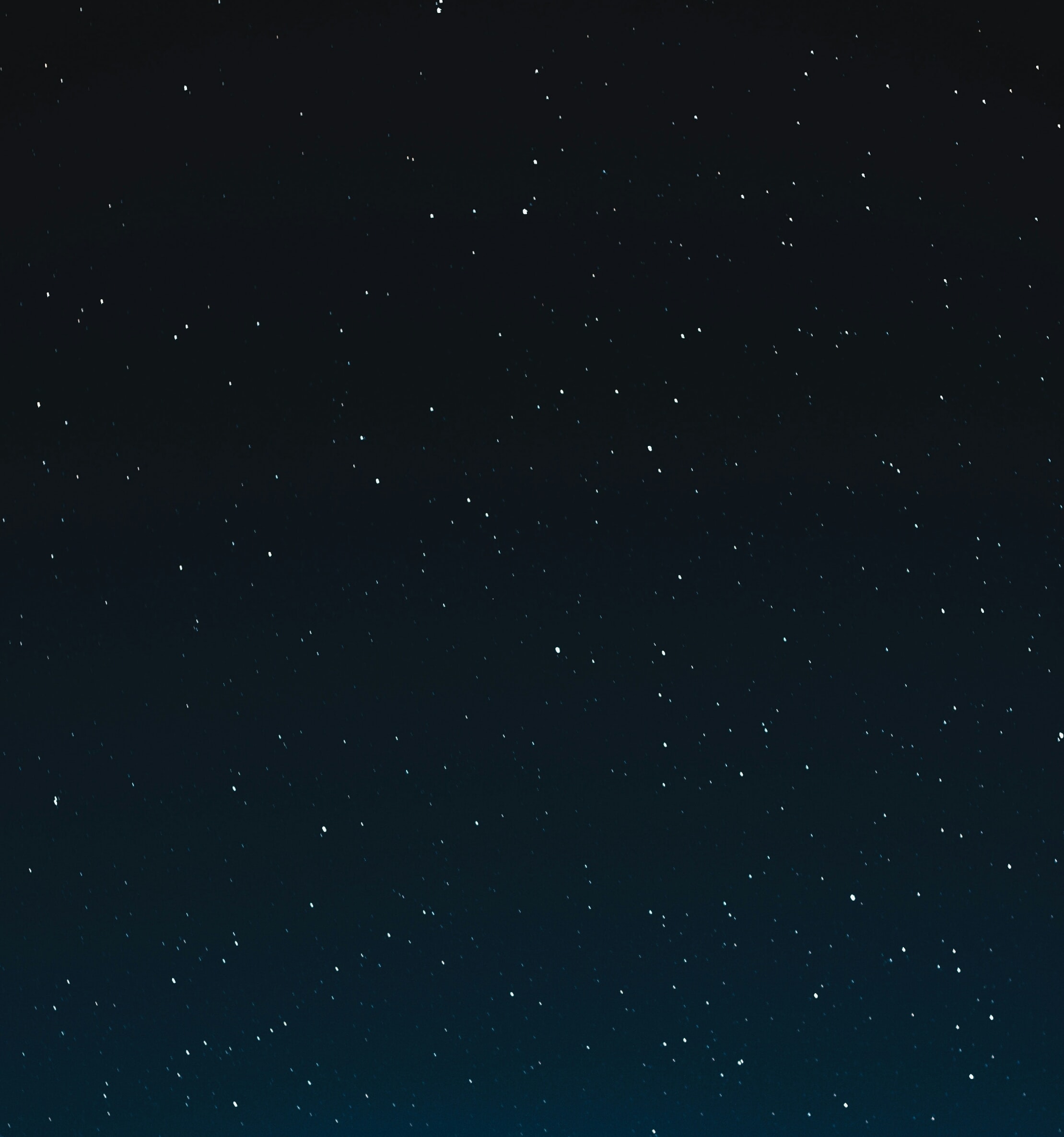
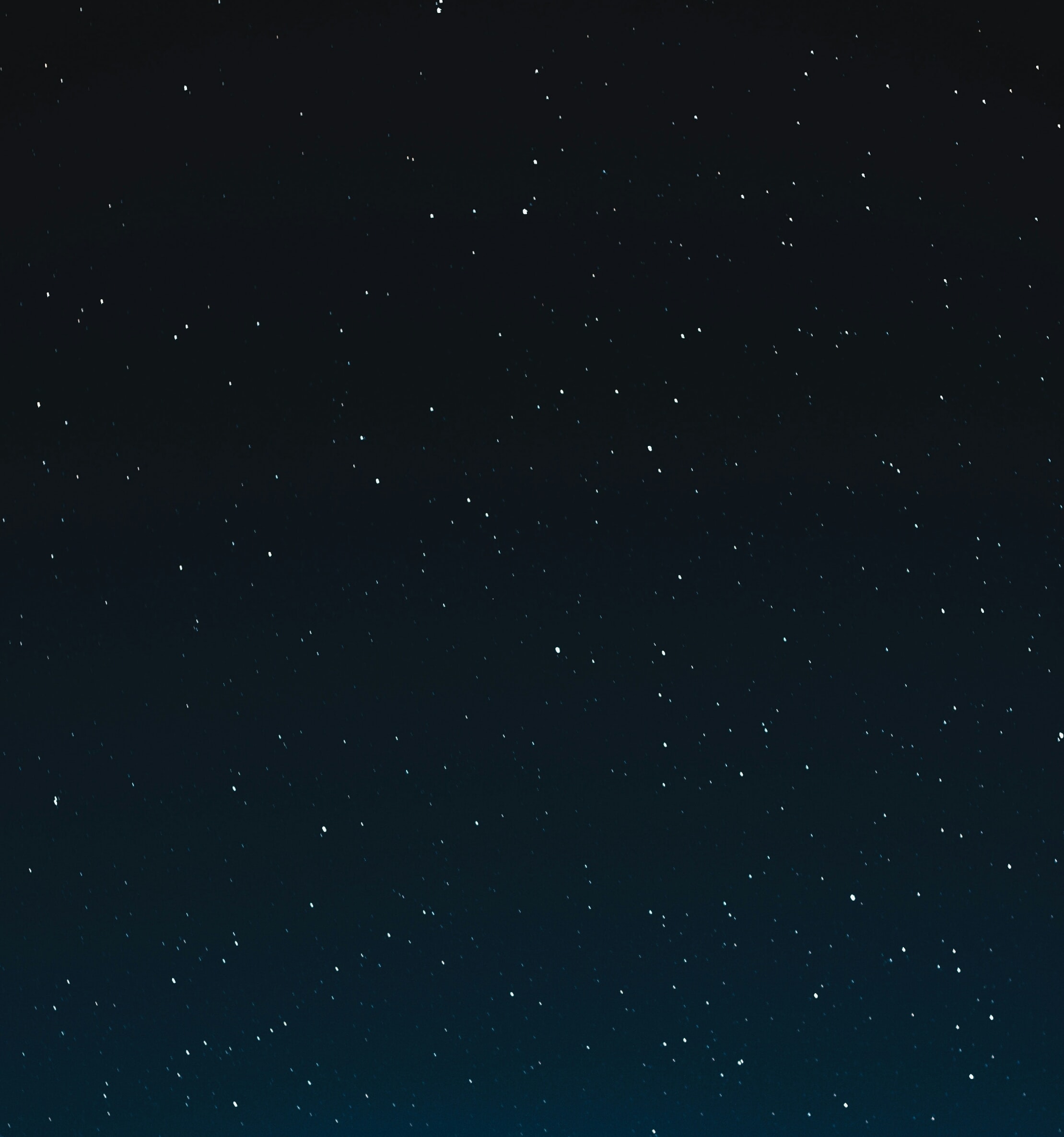
WHAT PROCESSES DRIVE LARGE–SCALE DISK STRUCTURES?
Project 1.2 | Lead: Kevin Wagner
Large-scale protoplanetary disk structures (e.g., annular gaps and spiral arms) have been identified in an increasing number of disks — in large part through studies led by our EOS Team — raising the question: What processes drive these structures? Understanding the nature of large-scale disk structures is key to understanding the processes of giant planet formation, which in turn affect the formation and future habitability of terrestrial planets. In at least one system, we now know of two giant planets that are driving large-scale disk structures and are readily accessible to direct studies. Our primary goal is to broaden the sample of disks with both imaged large-scale structures and imaged exoplanets, as these provide the best constraints on planet-disk interaction models. Second to this goal, we will enlarge the sample of disks with imaged large-scale structures to enable precise statistical constraints on parameters relevant to the formation of habitable-zone planets.
In Project 1.2 we will utilize the recently commissioned second-generation MagAO-X instrument for the first time to image large-scale structures in protoplanetary disks and, in some cases, to also image the protoplanets that are driving these structures.
THE COMPOSITIONS AND FORMATION HISTORIES OF PLANETESIMALS IN THE INNER SOLAR SYSTEM AND ON NEAR-EARTH ORBITS
Project 1.3 | Lead: Tom Zega
Pebble migration and accretion are key ingredients of modern planet-formation models. The efficient migration of pebbles from the outer to inner disks, however, typically leads to high abundances of carbon and oxygen in the inner disk region. This prediction is at odds with Earth’s very low water (<0.3 wt%) and carbon (<0.4 wt%) content. One solution to this contradiction is offered by disk substructures, possibly introduced by the formation of giant planets at large distances. Consistent with this idea is a hypothesis for Solar-System formation that relies on the early formation of Jupiter to block the inward drift of pebbles. Therefore, in this scenario, the relatively little carbon and water that Earth received were delivered much later by asteroids and comets (sometimes referred to as the “late veneer”) after the solar nebula’s dispersal. This hypothesis of Earth’s formation suggests that Earth-like compositions depend on the early formation of a giant planet an event that is probably not common. A key test for this hypothesis is understanding planetesimal oxygen (i.e., water) and carbon contents as a function of the location (within or beyond the orbit of Jupiter) and time (before or after Jupiter’s formation).
In Project 1.3 we will use a combination of laboratory-based techniques and millimeter-wave telescopic observations to test protoplanetary disk evolution models. Specifically, we will study the carbon and oxygen content of meteoritic and asteroid samples as well as that of comets to test the key processes responsible for carbon and oxygen processing in the inner and outer Solar System.
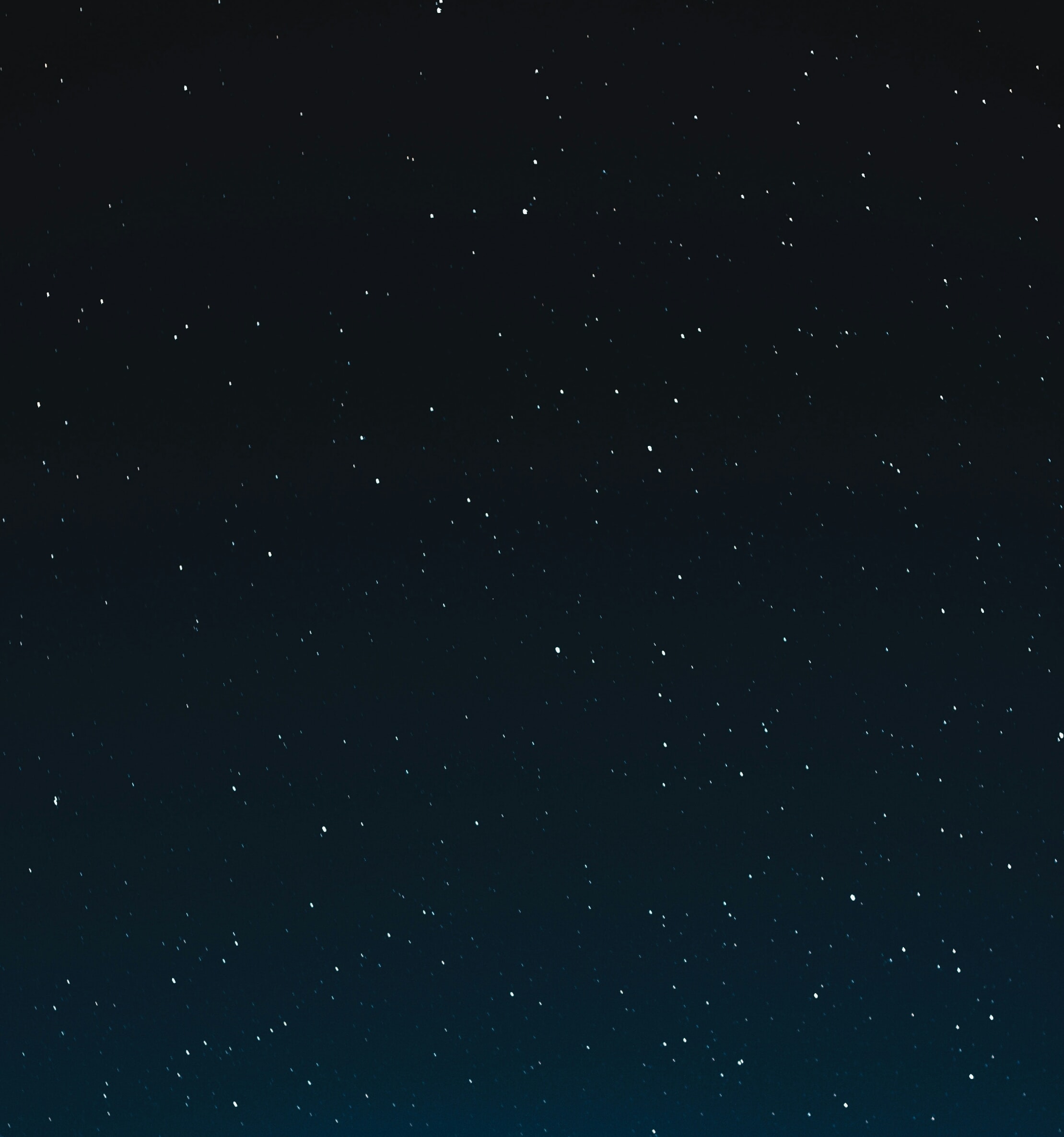
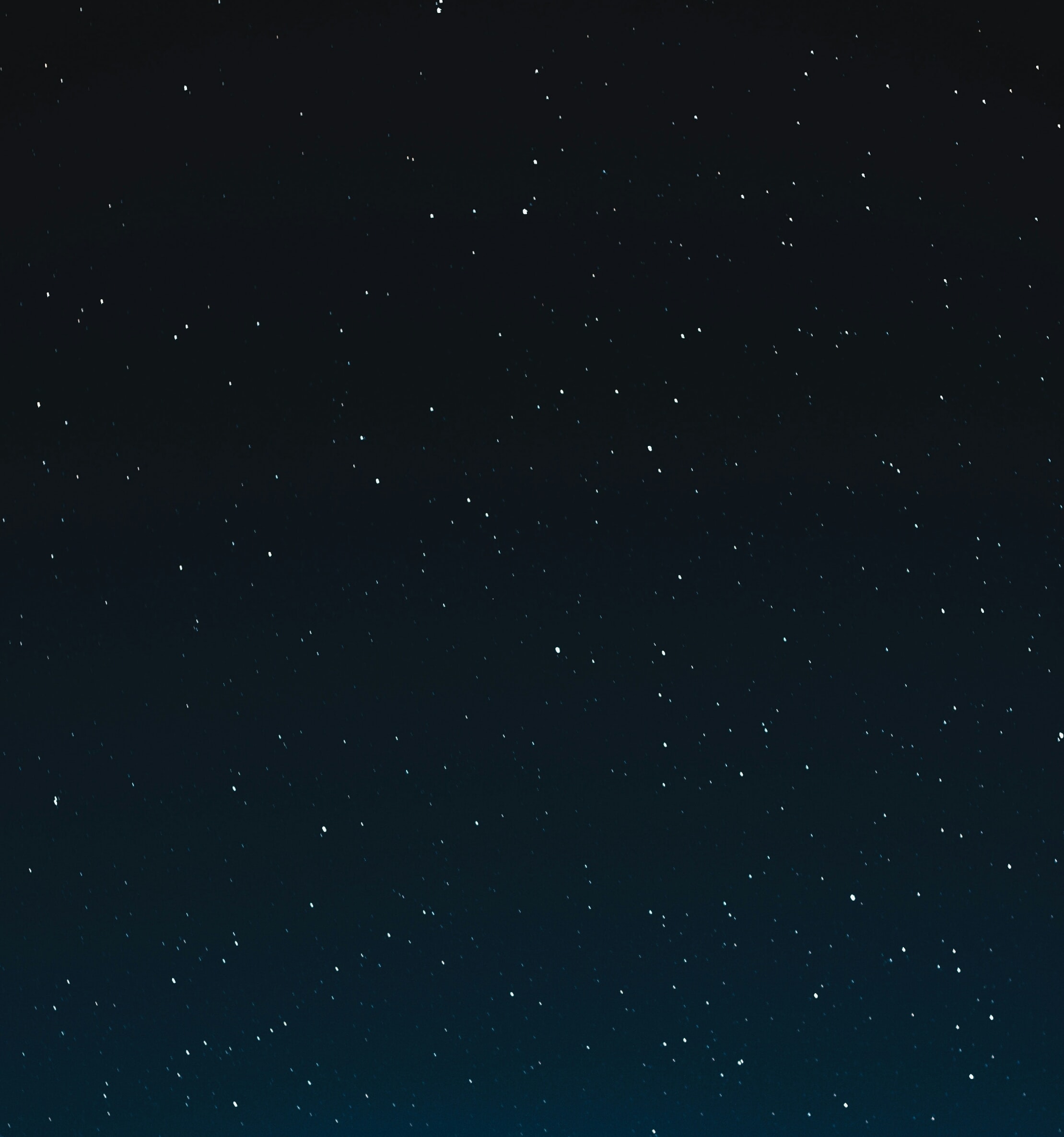
HOW DOES ONGOING GAS INFALL DURING THE EMBEDDED (CLASS I) PHASE AFFECT PLANET FORMATION AND DISK STRUCTURES?
Project 1.4 | Lead: Kaitlin Kratter
Kepler data has revealed an abundance of small, rocky planets, necessitating a revision of terrestrial planet formation models. Meanwhile, increasingly sensitive observations of protoplanetary disks at mm and cm wavelengths, coupled with sophisticated SED modeling, find primarily low solid disk masses compared to the minimum-mass solar nebula. This mismatch strongly suggests that grain growth, planetesimal formation, and perhaps even rocky core assembly begins in the Class I phase, while the protostellar envelope is still draining onto the disk. Moreover, high-resolution ALMA images have revealed many Class II disks with rings and gaps that might be produced by planets. These structures could indicate that the Class II disks are truly not “proto”planetary).
In Project 1.4 we will model the impact of ongoing infall on the pebble flux received by forming terrestrial planets. We will compare accretion rates onto inner planets in the presence and absence of wide orbit planets and their concomitant disk substructures.